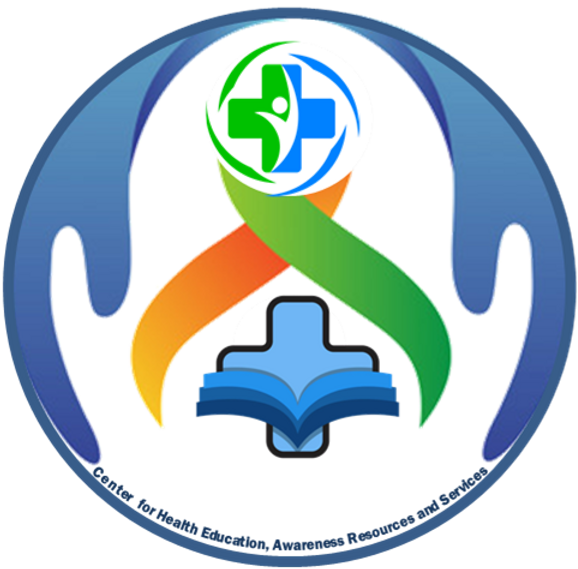

CHEARSpedia
Online publication portal of
Center for Health Education, Awareness Resources and Services
Open Journal of Health Research, Intervention and Awareness
Pub. ID :
82db8b1a-1aff-44dc-8fca-7f75b4c1c9f2
Section :
Awareness
ARTIFICIAL BLOOD: THE QUEST FOR SCIENCE’ S HOLY GRAIL
Arin Natania. S
Doctor of Pharmacy, Sri Ramakrishna Institute of Paramedical Sciences, Coimbatore
Ruban Charles
Bachelor of Engineering (Mech Dept.), CSI College of Engineering, Ketti, Nilgiris
ABSTRACT:
Artificial blood is a product made to act as a substitute for red blood cells. While true blood serves many different functions, artificial blood is designed for the sole purpose of transporting oxygen and carbon dioxide throughout the body. Depending on the type of artificial blood, it can be produced in different ways using synthetic production, chemical isolation, or recombinant biochemical technology. Development of the first blood substitutes dates back to the early 1600s, and the search for the ideal blood substitute continues. Various manufacturers have products in clinical trials; however, no truly safe and effective artificial blood product is currently marketed.
INTRODUCTION
Blood is a special type of connective tissue that is composed of white cells, red cells, platelets, and plasma. It has a variety of functions in the body. Plasma is the extracellular material made up of water, salts, and various proteins that, along with platelets, encourages blood to clot. Proteins in the plasma react with air and harden to prevent further bleeding. The white blood cells are responsible for the immune defense. They seek out invading organisms or materials and minimize their effect in the body.
The red cells in blood create the bright red color. As little as two drops of blood contains about one billion red blood cells. These cells are responsible for the transportation of oxygen and carbon dioxide throughout the body. They are also responsible for the “typing” phenomena. On the membranes of these cells are proteins that the body recognizes as its own. For this reason, a person can use only blood that is compatible with her type. Currently, artificial blood products are only designed to replace the function of red blood cells. It might even be better to call the products being developed now, oxygen carriers instead of artificial blood.
HISTORY
Other materials that were tried during the 1800s include hemoglobin and animal plasma. In 1868, researchers found that solutions containing hemoglobin isolated from red blood cells could be used as blood replacements. In 1871, they also examined the use of animal plasma and blood as a substitute for human blood. Both of these approaches were hampered by significant technological problems. First, scientists found it difficult to isolate a large volume of hemoglobin. Second, animal products contained many materials that were toxic to humans.
A significant breakthrough in the development of artificial blood came in 1883 with the creation of Ringer's solution—a solution composed of sodium, potassium, and calcium salts. This product evolved into a human product when lactate was added. While it is still used today as a blood-volume expander, Ringer's solution does not replace the action of red blood cells so it is not a true blood substitute.
Karl Landsteiner who has been called the father of immunology, determined that human beings could be separated into blood groups according to the capacity of their red cells to clot in the presence of different serums. He named his blood classification groups A, B, and O. A fourth group AB, was discovered the following year. The result of this work was that patient and donor could be blood-typed beforehand, making blood transfusion a safe and routine medical practice.
Blood transfusion research did not move forward until scientists developed a better understanding of the role of blood and the issues surrounding its function in the body. During World War I, a gum-saline solution containing galactoso-gluconic acid was used to extend plasma. If the concentration, pH, and temperature were adjusted, this material could be designed to match the viscosity of whole blood, allowing physicians to use less plasma..
In 1966, experiments with mice suggested a new type of blood substitute, perfluorochemicals (PFC). These are long chain polymers similar to Teflon. It was found that mice could survive even after being immersed in PFC. This gave scientists the idea to use PFC as a blood thinner. In 1968, the idea was tested on rats. The rat's blood was completely removed and replaced with a PFC emulsion. The animals lived for a few hours and recovered fully after their blood was replaced.
DESIGN
The ideal artificial blood product has the following characteristics. First, it must be safe to use and compatible within the human body. This means that different blood types should not matter when an artificial blood is used. It also means that artificial blood can be processed to remove all disease-causing agents such as viruses and microorganisms. Second, it must be able to transport oxygen throughout the body and release it where it is needed. Third, it must be shelf stable. Unlike donated blood, artificial blood can be stored for over a year or more. This is in contrast to natural blood which can only be stored for one month before it breaks down. There are two significantly different products that are under development as blood substitutes. They differ primarily in the way that they carry oxygen. One is based on PFC, while the other is a hemoglobin-based product.
Perfluorocarbons (PFC)
As suggested, PFC are biologically inert materials that can dissolve about 50 times more oxygen than blood plasma. They are relatively inexpensive to produce and can be made devoid of any biological materials. This eliminates the real possibility of spreading an infectious disease via a blood transfusion. From a technological standpoint, they have two significant hurdles to overcome before they can be utilized as artificial blood. First, they are not soluble in water, which means to get them to work they must be combined with emulsifiers—fatty compounds called lipids that are able to suspend tiny particles of perfluorochemicals in the blood. Second, they have the ability to carry much less oxygen than hemoglobin-based products. This means that significantly more PFC must be used. One product of this type has been approved for use by the Federal Drug Administration (FDA), but it has not been commercially successful because the amount needed to provide a benefit is too high. Improved PFC emulsions are being developed but have yet to reach the market.
Hemoglobin-based products
Hemoglobin carries oxygen from the lungs to the other tissues in the body. Artificial blood based on hemoglobin takes advantage of this natural function. Unlike PFC products where dissolving is the key mechanism, oxygen covalently bonds to hemoglobin. These hemoglobin products are different than whole blood in that they are not contained in a membrane so the problem of blood typing is eliminated. However, raw hemoglobin cannot be used because it would break down into smaller, toxic compounds within the body. There are also problems with the stability of hemoglobin in a solution. The challenge in creating a hemoglobin-based artificial blood is to modify the hemoglobin molecule so these problems are resolved. Various strategies are employed to stabilize hemoglobin. This involves either chemically cross-linking molecules or using recombinant DNA technology to produce modified proteins. Just as Polyethylene Glycol-Modified Liposome-Encapsulated Hemoglobin, nanoparticle and polymersome encapsulated hemoglobin, stabilized hemoglobin solutions, polymerized hemoglobin solutions, conjugated hemoglobin solutions.
Conjugation of hemoglobin effectively increases its molecular size and reduces antigenicity, resulting in a slow rate of removal from the circulation and reduced “visibility” to the reticuloendothelial system. Unique features of conjugated hemoglobins are their high oncotic pressure, which makes them very potent plasma-volume expanders, and their viscosity.
Intramolecular cross-linked hemoglobins are not significantly increased in molecular weight but have specific chemical cross-links between polypeptide chains that prevent dissociation to dimers or monomers.
These modified hemoglobins are stable and soluble in solutions. Theoretically, these modifications should result in products that have a greater ability to carry oxygen than our own red blood cells. It is anticipated that the first of these products will be available within one to two years.
RAW MATERIALS USED
Depending on the type of artificial blood that is made, various raw materials are used. Hemoglobin-based products can use either isolated hemoglobin or synthetically produced hemoglobin.
To produce hemoglobin synthetically, manufacturers use compounds known as amino acids. These are chemicals that plants and animals use to create the proteins that are essential for life. There are 20 naturally occurring amino acids that may be used to produce hemoglobin. All of the amino acid molecules share certain chemical characteristics. They are made up of an amino group, a carboxyl group, and a side chain. The nature of the side chain differentiates the various amino acids. Hemoglobin synthesis also requires a specific type of bacteria and all of the materials needed to incubate it. This includes warm water, molasses, glucose, acetic acid, alcohols, urea, and liquid ammonia.
For other types of hemoglobin-based artificial blood products, the hemoglobin is isolated from human blood. It is typically obtained from donated blood that has expired before it is used. Other sources of hemoglobin come from spent animal blood. This hemoglobin is slightly different from human hemoglobin and must be modified before being used.
MANUFACTURING PROCESS
The production of artificial blood can be done in a variety of ways. For hemoglobin-based products, this involves isolation or synthesization of hemoglobin, molecular modification then reconstitution in an artificial blood formula. PFC products involve a polymerization reaction. A method for the production of a synthetic hemoglobin-based product is outlined below.
Hemoglobin synthesis
To obtain hemoglobin, a strain of E. coli bacteria that has the ability to produce human hemoglobin is used. Over the course of about three days, the protein is harvested and the bacteria are destroyed. To start the fermentation process, a sample of the pure bacteria culture is transferred to a test tube that contains all the nutrients necessary for growth. This initial inoculation causes the bacteria to multiply. When the population is great enough, they are transferred to a seed tank. A seed tank is a large stainless steel kettle that provides an ideal environment for growing bacteria. It is filled with warm water, food, and an ammonia source which are all required for the production of hemoglobin. Other growth factors such as vitamins, amino acids, and minor nutrients are also added. The bacterial solution inside the seed tank is constantly bathed with compressed air and mixed to keep it moving. When enough time has passed, the contents of the seed tank is pumped to the fermentation tank. The fermentation tank is a larger version of the seed tank. It is also filled with a growth media needed for the bacteria to grow and produce hemoglobin. Since pH control is vital for optimal growth, ammonia water is added to the tank as necessary. When enough hemoglobin has been produced, the tank is emptied so isolation can begin. Isolation begins with a centrifugal separator that isolates much of the hemoglobin. It can be further segregated and purified using fractional distillation. This standard column separation met hod is based on the principle of boiling a liquid to separate one or more components and utilizes vertical structures called fractionating columns. From this column, the hemoglobin is transferred to a final processing tank.
Final processing
Here, it is mixed with water and other electrolyte to produce the artificial blood. The artificial blood can then be pasteurized and put into an appropriate packaging. The quality of compounds is checked regularly during the entire process. Particularly important are frequent checks made on the bacterial culture. Also, various physical and chemical properties of the finished product are checked such as pH, melting point, moisture content, etc. This method of production has been shown to be able to produce batches as large as 2,640 gal (10,000 L).
THE FUTURE
Currently, there are several companies working on the production of a safe and effective artificial blood substitute. The various blood substitutes all suffer from certain limitations. For example, most of the hemoglobin-based products last no more than 20-30h in the body. This compares to transfusions of whole blood that lasts 34 days. Also, these blood substitutes do not mimic the blood's ability to fight diseases and clot. Consequently, the current artificial blood technology will be limited to short-term blood replacement applications. In the future, it is anticipated that new materials to carry oxygen in the body will be found. Additionally, longer lasting products should be developed, as well as products that perform the other functions of blood.
REFERNCES
Sarkar S. Artificial blood. Indian J Crit Care Med. 2008;12(3):140-144. doi:10.4103/0972-5229.43685
Hemoglobin-Based Red Cell Substitutes. Robert M. Winslow. Johns Hopkins University Press, 1992.
Blood Substitutes--A Moving Target. Robert M. Winslow in Nature Medicine, Vol. 1, No. 11, pages1212-1215; 1995.
Blood Substitutes. Robert M. Winslow in Science & Medicine, Vol. 4, No. 2, pages 54-63; 1996.
7
90
read this
DOI :
10.6084/m9.figshare.14338838
Download Count :
7
Citation Count :
2
Dear Authors !
Please note that article submitted for CHEARSpedia should be either your review or research work. Rest all write-ups should be published on our CHEARS article page. As we are dedicated to the public health and awareness, we accept articles which has significant data to upgrade the knowledge of either general community or healthcare professional.